Dewyium: The Penultimate Atom
- Hunter Blain
- Jul 9, 2024
- 7 min read
Alchemy has had an interesting history through the years. Though the field has been re-branded into chemistry, there are still elements (pun intended) of its roots that can still be seen today. Indeed, science would not be where it is today without alchemical study.
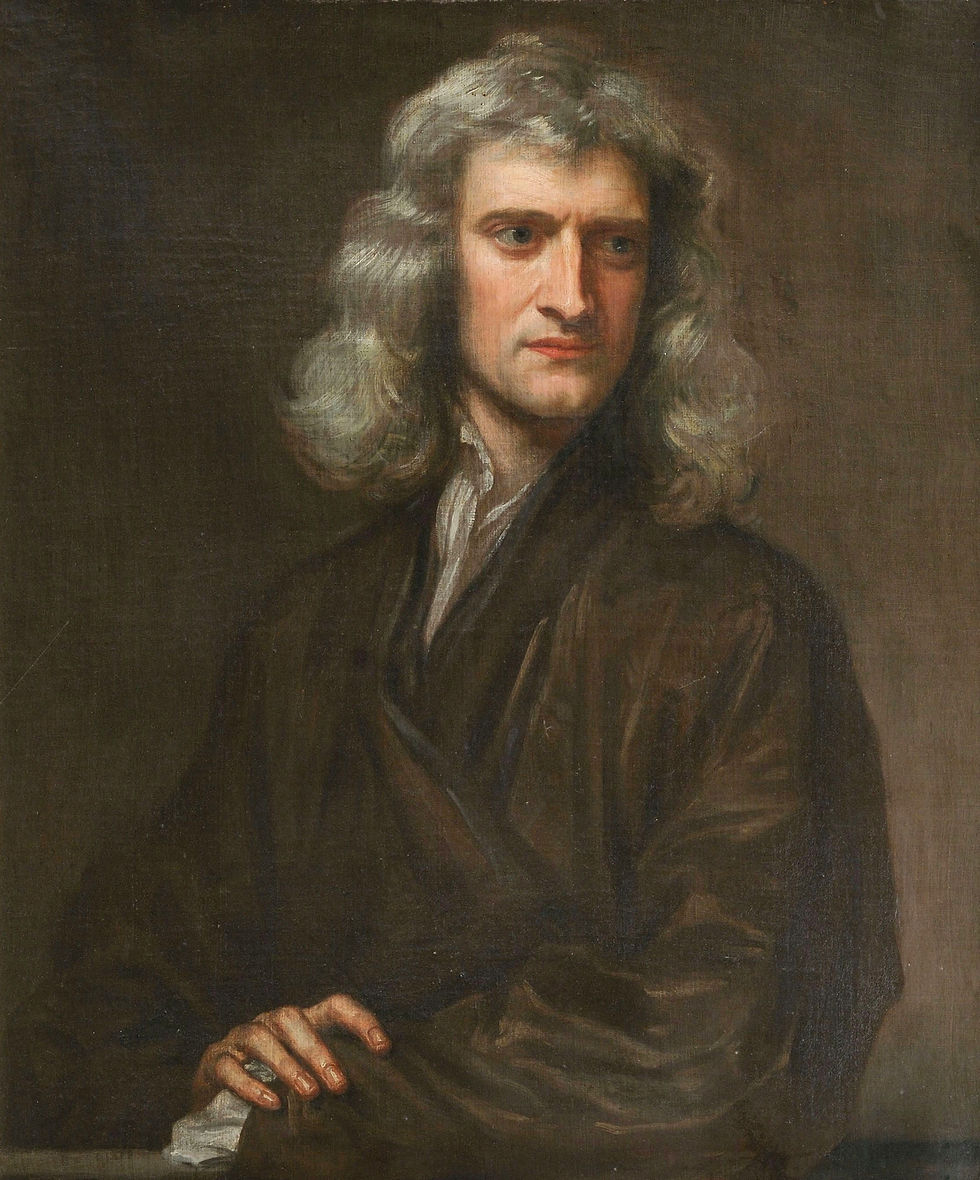
Pictured: Sir Issac Newton, inventor of calculus, quantifier of gravity and alchemist obsessed with finding the philosopher's stone.
If you want to check out some of his alchemical stuff, check out here.
I dabble in both science and magic. Honestly, I don't really see much of a meaningful distinction between the two. Recently I've been spending some time with a chemist's best friend: The Periodic Table.
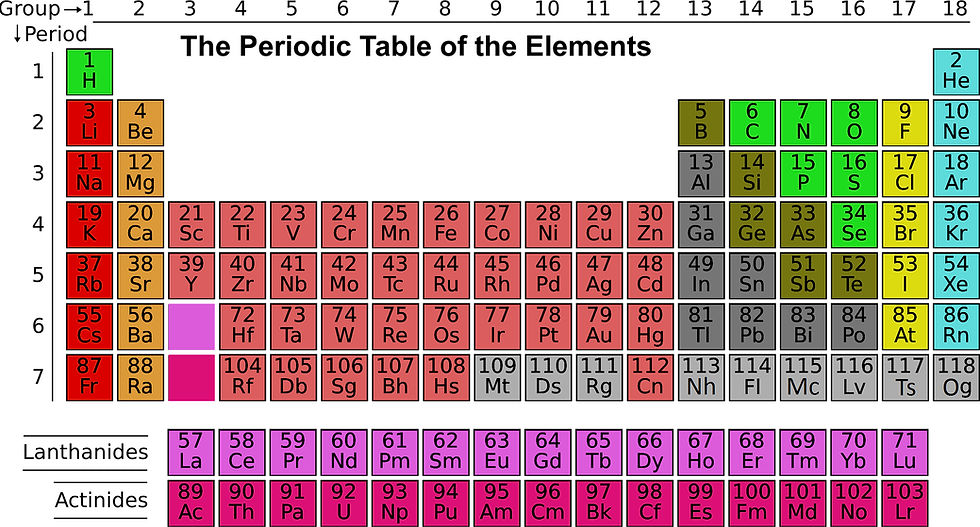
Pictured: This is the basic version that ends at Oganesson (atomic number 118). We will get into more detail later.
The periodic table has been a rolling project in chemistry since 1869, when Dimitri Mendeleev first started collecting and sorting elements in this manner. As researchers are able to play with the fabric of reality with more in-depth methods, scientists have been able to create heavier and heavier elements (typically by using a particle accelerator to smash atoms and subatomic particles into each other at unthinkable speeds). These elements are usually highly unstable, only lasting mere seconds before they decay into lighter elements. However, the discovery/creation of these new elements is an incredibly important scientific field. In addition to scientists being able to add their name or location to the vanity plates that are the names of heavier, non-naturally occurring elements (like Moscovium or Berkelium), it pushes the boundaries of what is thought possible.

Pictured: An extended version of the periodic table listing elements beyond 118, as proposed by Andrey Kulsha.
As of the publishing of this article, there have been multiple attempts to create atoms beyond 118 (which was discovered in 2006), but none have been successful. However, there is no reason to believe that people will stop in this pursuit. As time progresses, it seems inevitable that more and more elements will be created/discovered. I have neither the time, expertise, credentials nor resources to join this group of scientists.
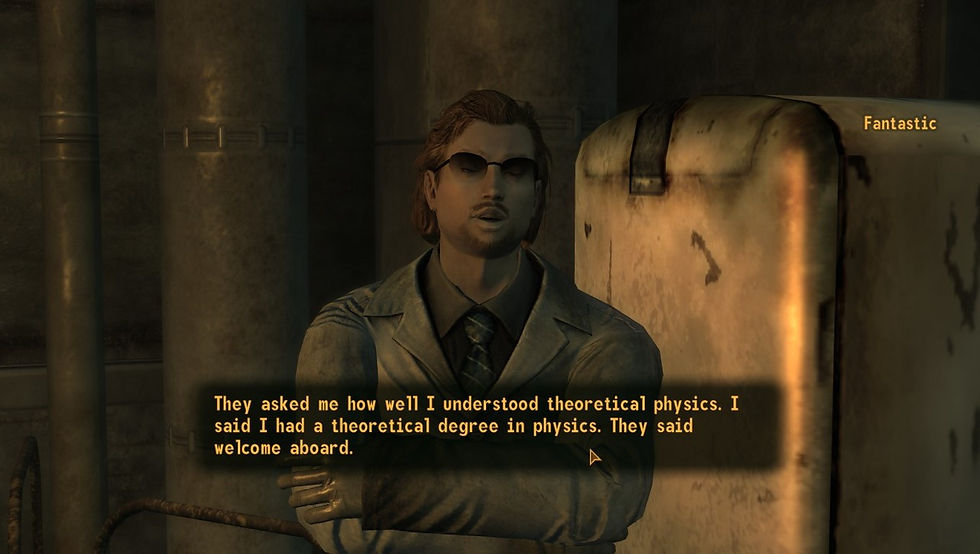
Pictured: My qualifications.
But in all seriousness, let's dive into theoretical nuclear physics/chemistry!
However, I want to ask a bit of a different, more conceptual question. Is there a limit to how far down atomic numbers can go? Is there a point that, if you attempt to create a heavier elements, physics break?
I'm hardly the first person to come up with this problem. I've found multiple other scholarly articles (example, another example) asking a very similar question. However, they are all behind paywalls because that's how science works now and I don't exactly have a ton of money to throw at this (they want like $40/article and there are too many that fit the bill). So all I can see from my broke point of view is their abstracts.
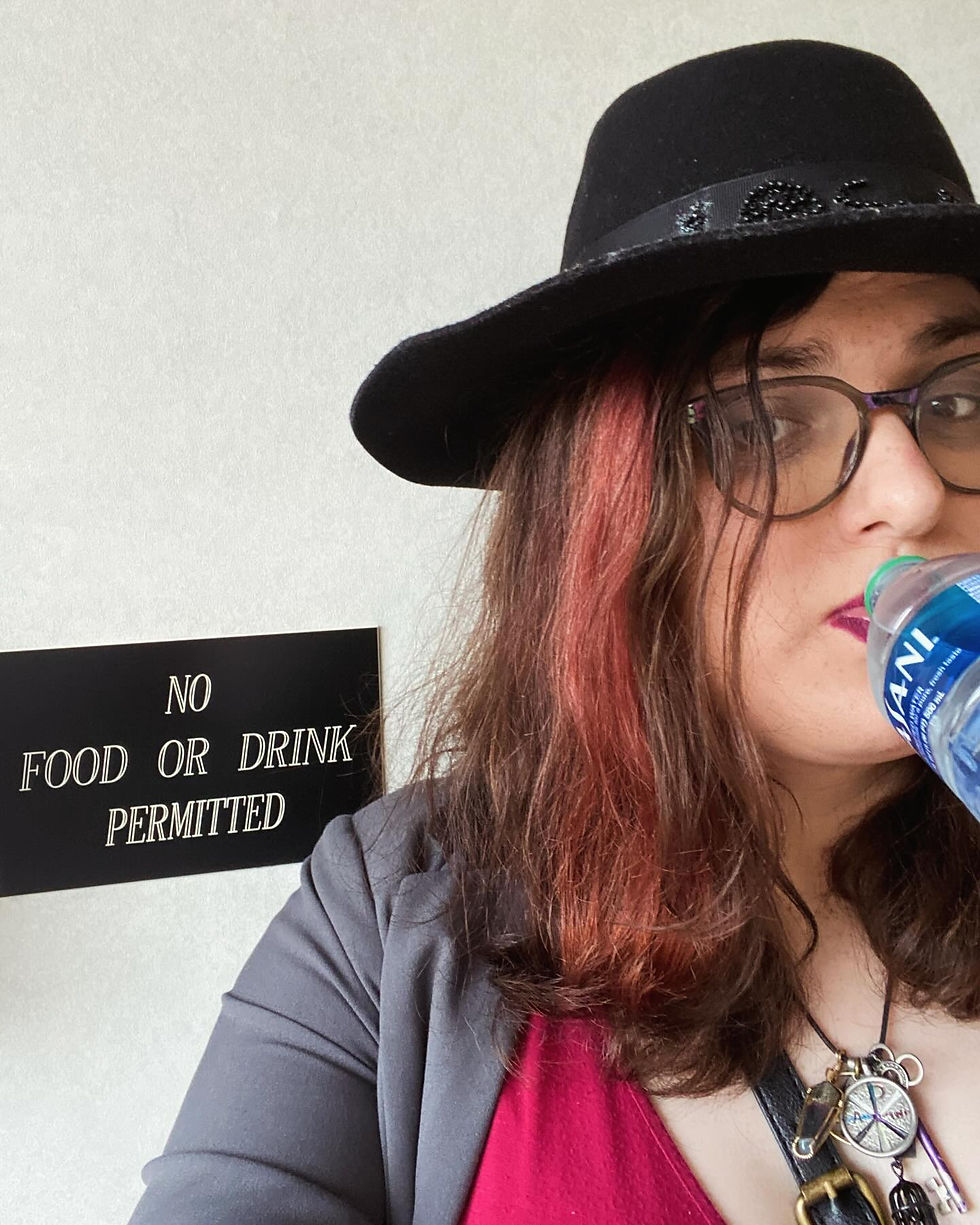
Pictured: I, on the other hand, publish the results of my scientific ventures freely. Just saying. *sips tea*
From these abstracts, I'm only seeing discussions of the problem and the current science around it instead of the direction I will be taking this in. The abstracts note that there will be "future challenges" and speculate about "future directions" in the field. But they notably do NOT say that they have a prediction of what will inevitably occur far enough down the atomic line. (If they do, then everyone in this field sucks at writing a comprehensive abstract, which seems unlikely given the sheer number of articles I encountered).
This is also somewhat typical of scientific inquiry at this time; researchers show an unwillingness to take a definitive stab at things without prior things to point to. It's not their fault; it's how they are taught. So, often, the "conclusion" of these papers is something like: "Well, we don't know except for an atom-by-atom basis and will have to see as it happens." It's an academically safe way to proceed in a manner that doesn't upset the status quo, but that's not really my style.
But enough about me yammering on about my problems with the current state of scientific research and back to the problem at hand!
To better explain what I am getting at, let's take a quick refresher on the structure of the atom. We don't really need to get into quarks and subatomic things for the purposes of this article, so this is going to be pretty basic.
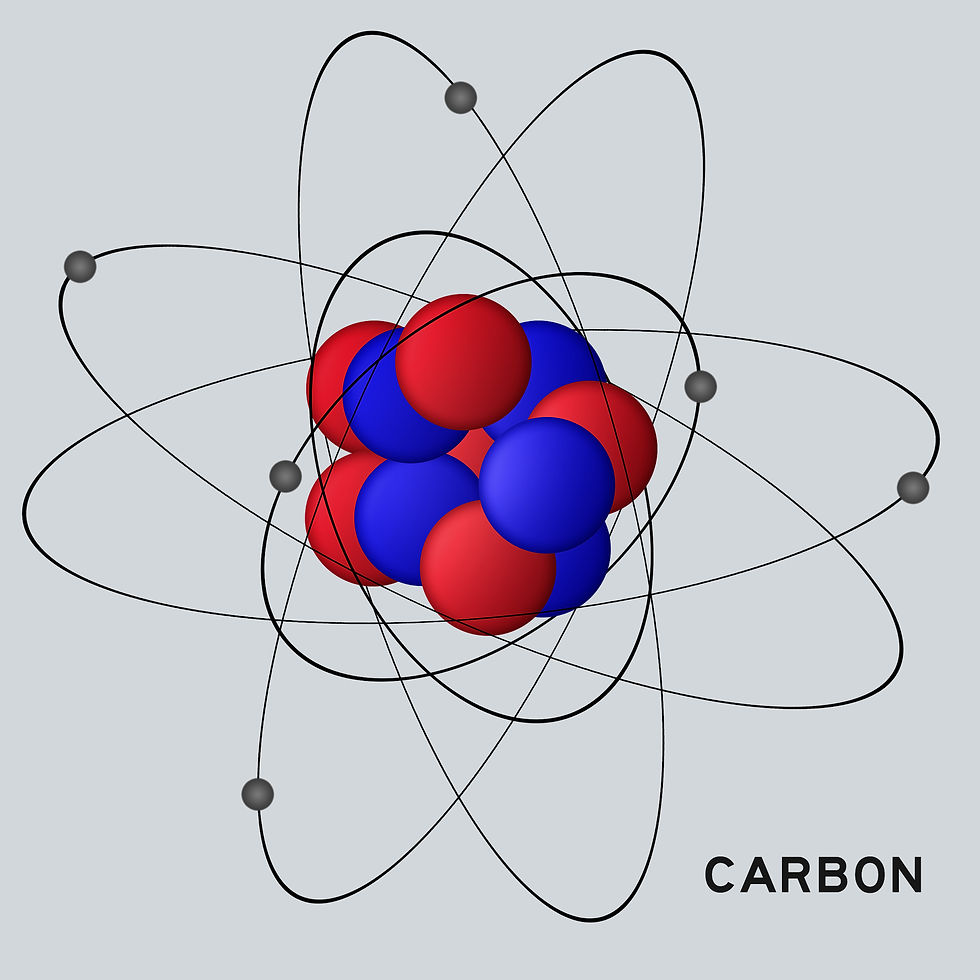
Pictured: A carbon atom, which has six protons and six electrons (the normal amount of neutrons is six as well, but a there are other isotopes of carbon with seven and eight neutrons; this is what carbon dating is based on).
There are three primary kinds of atomic particles: protons, neutrons and electrons. Protons have a positive charge, electrons have a negative charge and neutrons have no charge and just add mass to the atom. If the number of protons to electrons is not even, you don't have an atom, you have an ion (which can be charged negatively or positively, depending on how the imbalance resolves).
Protons and neutrons are in the center of the atom (called the nucleus), where they live close together. Electrons orbit the nucleus within the atom's electron cloud (an area where the electrons can be). The electron cloud has a far larger diameter than the above picture of a carbon atom above suggests. For example, a typical hydrogen atom is an incredibly simple atom with one proton and one electron. The atom's total diameter, including the electron cloud, is roughly 25000 times larger than the lone proton in the nucleus. So, if that proton was the size of a basketball, the electron cloud would be roughly the size of a small city.
Without spoiling too much, the tree I am barking up concerns mass and density. Protons technically weigh a little less than neutrons but both weigh approximately one atomic mass unit (also known as a Dalton). Electrons weigh 1/1836th of a proton. To give a sense of scale, a Dalton is equal to roughly 1.66053892173 times 10 to the negative 24th power. So, one Dalton is equal to 0.00000000000000000000000166053892173 grams. An electron is significantly lighter than a Dalton, at 9.04432963905 times 10 to the negative 28th power (0.000000000000000000000000000904432963905 grams).
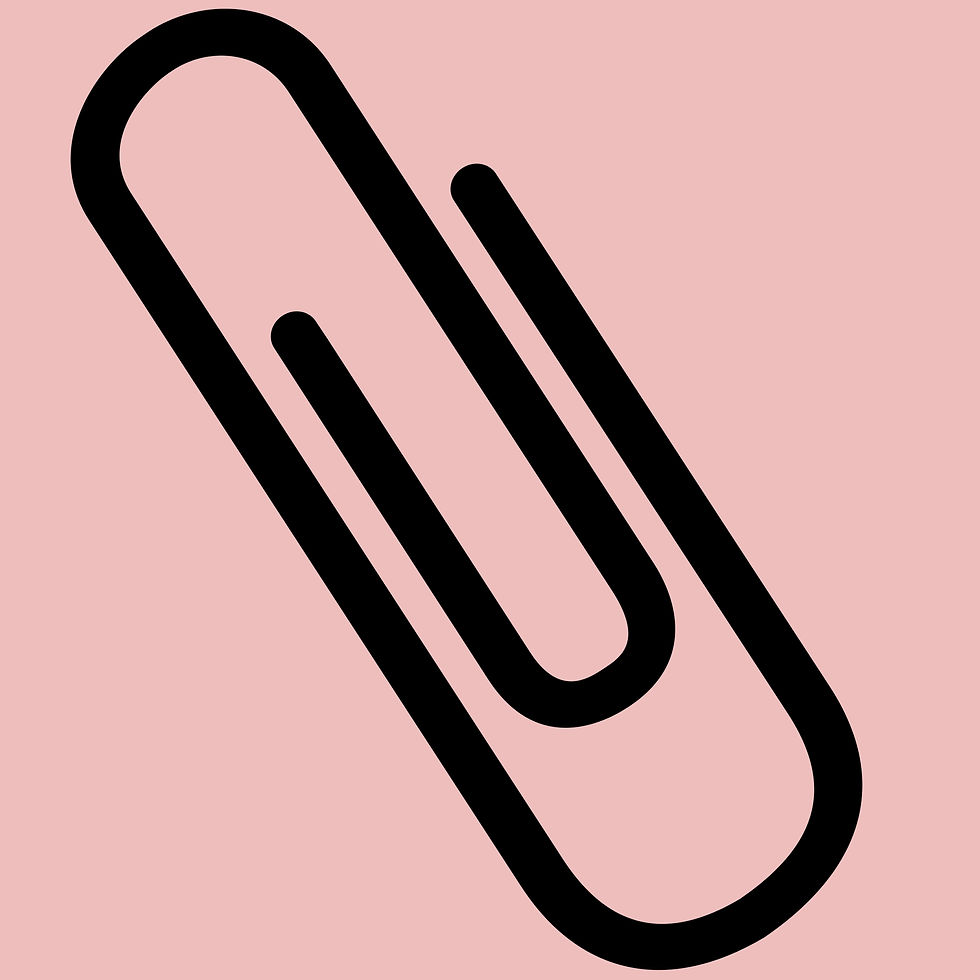
Pictured: A normal paperclip weighs about one gram. It's hardly news that atoms don't weigh much, but it's still pretty cool to scale things.
Okay. I think the stage is set. Enough basic nerd stuff. Now to more advanced nerd stuff.
The vast majority of atoms (and, by extension, matter) is empty space held in place by relative charge instead of being physically adjacent. However, an atom's density is not distributed equally within the atom; the vast majority of mass is found in the nucleus. Thus, the nuclear density of an atom is leagues more dense than the atom overall. As we go down the periodic table, the weight of the tightly packed nucleus continues to grow. Indeed, these are the densest (albeit tiny) areas of the universe we know.
At a certain point, once an object becomes dense enough, it will collapse into a black hole (thanks Einstein). Figuring out the exact point beforehand where this would happen is likely an impossible task (or maybe I just don't know how to calculate this; see my qualifications above). We do know that the smaller a black hole is, the more dense it has to be for the collapse to occur.
But, at some point, this would happen. Given research and science's ever-present march, I theorize that this point will be reached... eventually. Not exactly precise, but that's the nature of things. I'm going to call the heaviest theoretical atom that can be made without creating a black hole Dewyium and the lightest theoretical atom that will result in a black hole Jesusium (because if you directly encounter this element, that's who you are going to meet).
Though we cannot calculate the atomic number of Dewyium or Jesusium, we do know enough about black holes to speculate how these created black holes would behave.
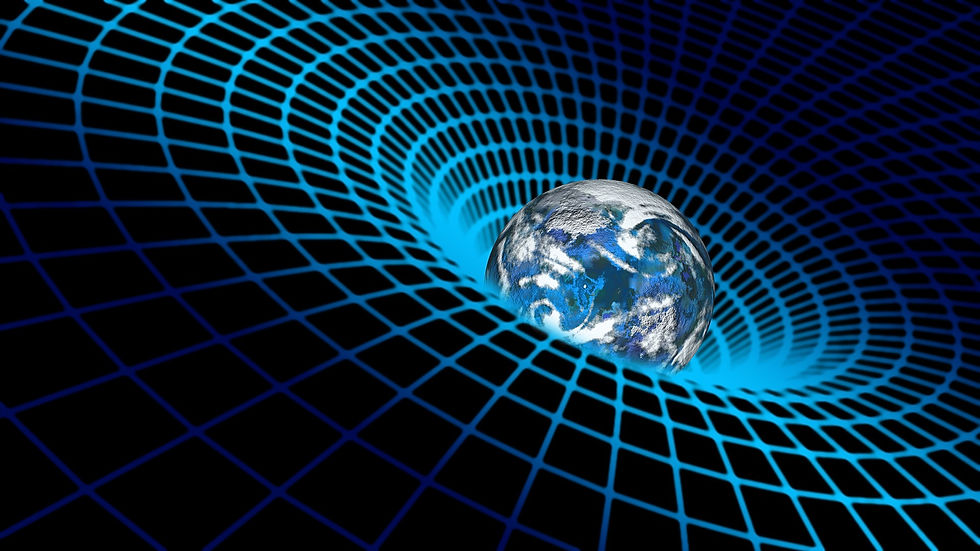
Pictured: A principle of general relativity is that mass bends spacetime around it in proportion to how massive that object is.
First, black holes slowly lose mass over time via a process called Hawking radiation. Basically, at the edge of a black hole is what is called the "event horizon." Once an object passes this threshold, there is no way for it to escape the incredible gravity of the black hole (not even light).
As a black-body object, black holes do thermally radiate. However, any radiation that originates from within the event horizon simply will get sucked back in and reincorporated in the singularity at the center of a black hole. On the other hand, any of the radiation that emanates from beyond the event horizon will escape. Over a long period of time, this will wear down the black hole until it finally dissipates. In other words, black holes do have a lifespan (which is extremely long and on a cosmic scale, but still finite).
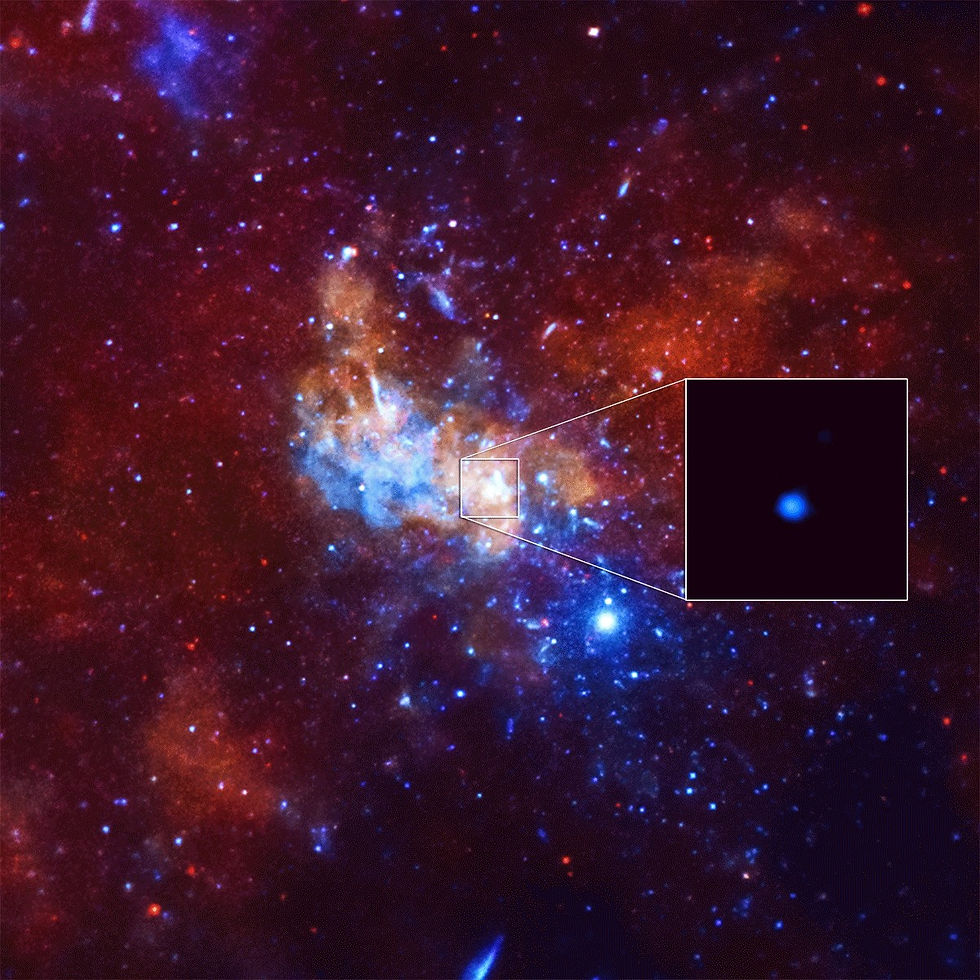
Pictured: An X-Ray flare from Sagittarius A, the supermassive black hole at the center of our galaxy.
Hawking radiation happens at an incredibly small scale, so larger black holes (such as the supermassive black hole that lives in the center of the Milky Way) will take such a long time to dissipate that it doesn't really mean anything. However, a tiny, atomic-sized black hole that Jesusium would create is so tiny that the black hole would radiate away its mass very quickly. So, unless the black hole was fed more mass, the black hole would dissipate nearly instantaneously.
You'll be happy to know that, in addition to being very short lived, a black hole with the mass of a heavy atomic nucleus wouldn't accidentally suck in the entire planet and kill us all (at least, in theory). In fact, you would be safe from its effects if you were merely meters away.
Being able to create black holes of any scale (and survive) would represent an incredible milestone in scientific achievement. And it seems that is the path we are currently on. We truly do live in amazing times.
One final observation: It is almost certain that Dewyium will be created before Jesusium. However, scientists have no way of knowing they've created Dewyium until they have created Jesusium. But, once that atom has been created, we will finally have reached the end of the periodic table.